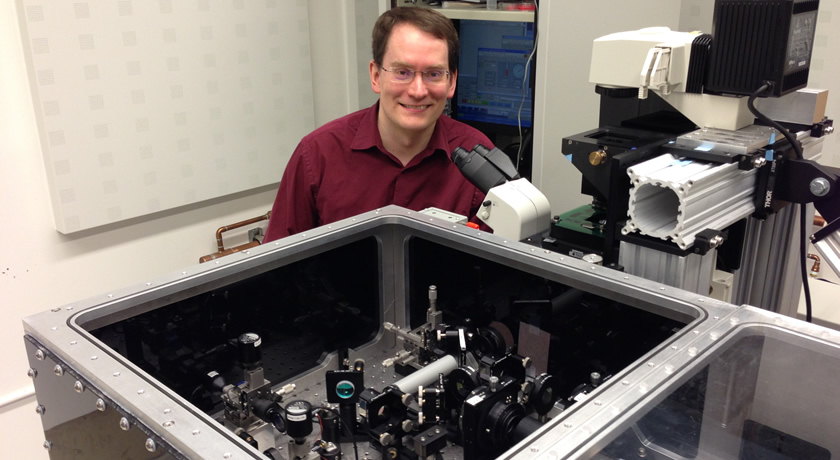
Michael Woodside with the apparatus he build to observe how protein molecules self-assemble. Proteins that "misfold" into the wrong structures are associated with diseases such as Parkinson's, ALS, Creutzfeld-Jakob, and Mad Cow. Photo: Suzette Chan.
A University of Alberta research group's new findings about molecular folding have recently been featured in two prestigious science journals.
The group is led by Michael Woodside, an associate professor of physics, who studies the physics of folding in biomolecules. The first author of both papers is Krishna Neupane, a research associate in Woodside's group.
Molecules like proteins, DNA, and RNA self-assemble into complex structures. Understanding how this "folding" works is important because of the link between structure, function, and disease. For example, proteins that "misfold" into the wrong structures are associated with diseases such as Parkinson's, ALS, Creutzfeld-Jakob, and Mad Cow.
Using specialized equipment for manipulating and studying single biological molecules, Woodside's group became the first scientists to see the motions of single molecules during the critical, but very brief, moments in which the structure actually changes. These findings were published by the journal, Science.
"In folding, we're interested in how things change from one structure to another," says Woodside. "We usually only see the beginning and end states because the states in between exist for such short periods of time."
Scientists have had to deduce the nature of those "invisible states" from changes in the measurable properties of the molecule before and after folding. But Woodside's state-of-the-art optical tweezers at the National Institute for Nanotechnology use light to trap and observe molecules with sufficient resolution to observe the microscopic motions. "We can see what's happening down to a bit below 10 microseconds; we're aiming for one microsecond, to see even more detail."
Woodside says, "The significance of the findings are in being able to see the transitional states directly for the first time and allowing us to test experimentally the microscopic physics of folding." Previously, the transitional states could only be seen in computational simulations.
The second paper, published in Nature Physics, explored another aspect of the folding problem: does the 30-year old theory of protein folding describe what's observed experimentally? A completely experimental test of the theory had not been done yet.
"This study started off with a different goal," says Woodside. "It started with a technical question about whether the way we've been looking at folding is a good way of doing it."
Woodside's group applies tension along the length of a molecule (a protein molecule is a long string) with the optical tweezers, but that technique measures just one experimental dimension. Woodside used ideas from chemistry and methods from theory and computation to devise statistical tests to assess the validity of how the group measures.
"We found that it is indeed possible to describe protein folding properly through this technique," Woodside says. "In fact, we were able to go beyond this question and show that what we see matches quantitatively with what is expected from the physical theory of folding, providing a fully experimental validation of the theory."